Einstein’s latest anniversary marks the birth of modern cosmology
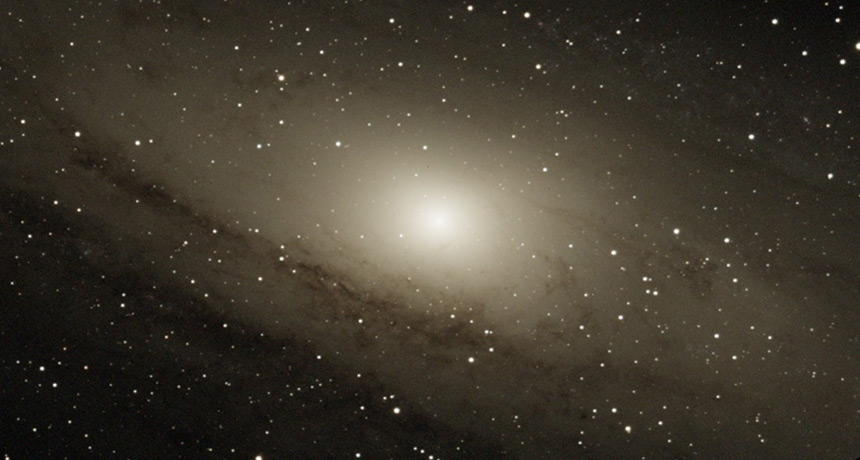
First of two parts
Sometimes it seems like every year offers an occasion to celebrate some sort of Einstein anniversary.
In 2015, everybody lauded the 100th anniversary of his general theory of relativity. Last year, scientists celebrated the centennial of his prediction of gravitational waves — by reporting the discovery of gravitational waves. And this year marks the centennial of Einstein’s paper establishing the birth of modern cosmology.
Before Einstein, cosmology was not very modern at all. Most scientists shunned it. It was regarded as a matter for philosophers or possibly theologians. You could do cosmology without even knowing any math.
But Einstein showed how the math of general relativity could be applied to the task of describing the cosmos. His theory offered a way to study cosmology precisely, with a firm physical and mathematical basis. Einstein provided the recipe for transforming cosmology from speculation to a field of scientific study.
“There is little doubt that Einstein’s 1917 paper … set the foundations of modern theoretical cosmology,” Irish physicist Cormac O’Raifeartaigh and colleagues write in a new analysis of that paper.
Einstein had pondered the implications of his new theory for cosmology even before he had finished it. General relativity was, after all, a theory of space and time — all of it. Einstein’s showed that gravity — the driving force sculpting the cosmic architecture — was simply the distortion of spacetime geometry generated by the presence of mass and energy. (He constructed an equation to show how spacetime geometry, on the left side of the equation, was determined by the density of mass-energy, the right side.) Since spacetime and mass-energy account for basically everything, the entire cosmos ought to behave as general relativity’s equation required.
Newton’s law of gravity had posed problems in that regard. If every mass attracted every other mass, as Newton had proclaimed, then all the matter in the universe ought to have just collapsed itself into one big blob. Newton suggested that the universe was infinite, filled with matter, so that attraction inward was balanced by the attraction of matter farther out. Nobody really bought that explanation, though. For one thing, it required a really precise arrangement: One star out of place, and the balance of attractions disappears and the universe collapses. It also required an infinity of stars, making it impossible to explain why it’s dark at night. (There would be a star out there along every line of sight at all times.)
Einstein hoped his theory of gravity would resolve the cosmic paradoxes of Newtonian gravity. So in early 1917, less than a year after his complete paper on the general theory was published, he delivered a short paper to the Prussian Academy of Sciences outlining the implications of his theory for cosmology.
In that paper, titled “Cosmological Considerations in the General Theory of Relativity,” he started by noting the problems posed by using Newton’s gravity to describe the universe. Einstein showed that Newton’s gravity would require a finite island of stars sitting in an infinite space. But over time such a collection of stars would evaporate. That problem could be avoided, though, if the universe turned out not to be infinite. Instead, Einstein said, everything would be fine if the universe is finite. Big, sure, but curved in such a way that it closed on itself, like a sphere.
Einstein’s mathematical challenge was to show that such a finite cosmic spacetime would be static and stable. (In those days nobody knew that the universe was expanding.) He assumed that on a large enough scale, the distribution of matter in this universe could be considered uniform. (Einstein said it was like viewing the Earth as a smooth sphere for most purposes, even though its terrain is full of complexities on smaller distance scales.) Matter’s effect on spacetime curvature would therefore be pretty much constant, and the universe’s overall condition would be unchanging.
All this made sense to Einstein because he had a limited view of what was actually going on in the cosmos. Like many scientists in those days, he believed the universe was basically just the Milky Way galaxy. All the known stars moved fairly slowly, consistent with his belief in a spherical cosmos with uniformly distributed mass. Unfortunately, general relativity’s math didn’t work if that was the case — it suggested the universe would not be stable. Einstein realized, though, that his view of the static spherical universe would succeed if he added a term to his original equation.
In fact, there were good reasons to include the term anyway. O’Raifeartaigh and colleagues point out that in his earlier work on general relativity, Einstein remarked in a footnote that his equation technically permitted the inclusion of an additional term. That didn’t seem to matter at the time. But in his cosmology paper, Einstein found that it was just the thing his equation needed to describe the universe properly (as Einstein then supposed the universe to be). So he added that factor, designated by the Greek letter lambda, to the left-hand side of his basic general relativity equation.
“That term is necessary only for the purpose of making possible a quasi-static distribution of matter, as required by the fact of the small velocities of the stars,” Einstein wrote in his 1917 paper. As long as the magnitude of this new term on the geometry side of the equation was small enough, it would not alter the theory’s predictions for planetary motions in the solar system.
Einstein’s 1917 paper demonstrated the mathematical effectiveness of lambda (also called the “cosmological constant”) but did not say much about its physical interpretation. In another paper, published in 1918, he commented that lambda represented a negative mass density — it played “the role of gravitating negative masses which are distributed all over the interstellar space.” Negative mass would counter the attractive gravity and prevent all the matter in Einstein’s spherical finite universe from collapsing.
As everybody now knows, though, there is no danger of collapse, because the universe is not static to begin with, but rather is rapidly expanding. After Edwin Hubble had established such expansion, Einstein abandoned lambda as unnecessary (or at least, set it equal to zero in his equation). Others built on Einstein’s foundation to derive the math needed to make sense of Hubble’s discovery, eventually leading to the modern view of an expanding universe initiated by a Big Bang explosion.
But in the 1990s, astronomers discovered that the universe is not only expanding, it is expanding at an accelerating rate. Such acceleration requires a mysterious driving force, nicknamed “dark energy,” exerting negative pressure in space. Many experts believe Einstein’s cosmological constant, now interpreted as a constant amount of energy with negative pressure infusing all of space, is the dark energy’s true identity.
Einstein might not have been surprised by all of this. He realized that only time would tell whether his lambda would vanish to zero or play a role in the motions of the heavens. As he wrote in 1917 to the Dutch physicist-astronomer Willem de Sitter: “One day, our actual knowledge of the composition of the fixed-star sky, the apparent motions of fixed stars, and the position of spectral lines as a function of distance, will probably have come far enough for us to be able to decide empirically the question of whether or not lambda vanishes.”